Abstract:
Background:
Although the use of calcium sulfate has been used as a bone
graft substitute in the treatment of fractures for more than a
century, few studies investigate the use of recently developed
injectable calcium sulfate-based bone graft substitute with high
compressive strength after curing in situ. In this study,
we evaluated preliminary results of a high compressive strength
calcium sulfate-based bone graft substitute used in the
treatment of periarticular fractures.
Methods:
Sixty-one patients with periarticular fractures who were treated
with an injectable biodegradeble calcium sulfate cement (BCSC) (
MIIG X3, Wright Medical Technology, Inc, Arlington, TN, USA)
between October 2005 and
June 2008
were included in this study. The fracture location distribution
was as follows: Four proximal humeral fractures, four acetabular
fractures, thirty-six tibial plateau fractures, seven distal
tibial fractures and ten calcaneal fractures. Postoperative
roentgenologic study was adapted to evaluate the congruity of
the articular surface, bone replacement profile and the
resorption process of the bone substitute. The postoperative
joint function recovery assessment was achieved with Neer’s
score system, modified D’Aubigne and Postel hip scale,
Rasmussen’s functional and anatomical grading score system and
Mazur ankle score correspondingly to the shoulder, hip, knee and
ankle joints. The Maryland Foot Score Profile was adapted for
the postoperative evaluation of calcaneal fractures.
Results:
Fifty-seven of the 61 patients were followed-up successfully.
The average length of follow-up was 20.2
months (range:
12-27months).
Fractures healed uneventfully in all patients without infection
. According to the corresponding functional score system in each
location, joint function was excellent or good. Two patients
with tibial plateau fractures had an articular subsidence of 2mm
showed by X-ray film one year after operation without joint
dysfunction. Four weeks after surgery, partial absorption of
BCSCwas evident on radiographs. Six months postoperatively,
radiographs showed areas previously filled with BCSC with the
same bone density as normal cancellous bone.
Conclusion:
This study showed that use of a high compressive strength
calcium sulfate-based bone graft substitute to fill metaphyseal
defects in the treatment of periarticular fractures can provide
adequate immediate stability and strength for fracture reduction
while improving the safety of early stage joint motion in
functional rehabilitation.
J.Orthopaedics 2009;6(3)e14
Keywords:
Evaluation of high compressive strength calcium sulfate; bone
graft
Introduction:
Despite many advances in the management of fractures,
periarticular fractures continue to be a great challenge to
orthopaedic surgeons because of articular
surface depression and compaction into the subchondral
cancellous bone. To acquire optimal joint function, intra-articular
fracture fragments must be anatomically reduced to guarantee the
congruity of the articular surface, and initial stability is
necessary for early stage rehabilitation. After articular
surface reduction, the periarticular metaphysis is often left
with significant bone loss. This intraosseous void may need to
be filled with a bone graft to provide mechanical support to the
articular surfaces during healing.
Autogenous bone, typically harvested from the iliac crest,
traditionally remains the standard of bone grafting. It can be
associated, however, with an inadequate amount of material and
donor-site morbidity, including chronic pain and wound
complications[1]
[2].
Alternative graft materials for filling fracture voids include
allograft bone and synthetic bone graft substitute. While the
use of allograft avoids the donor-site morbidity associated with
the use of autograft, it may also lead to complications,
including potential disease transmission, slower incorporation,
and possibly lower union rates[3,4]
[5]
[6].
Therefore, structural bone graft substitutes, such as calcium
sulphate, calcium phosphate, porous coralline ceramics and
tricalcium phosphate, appear to be an attractive alternative
because they lack the disadvantages of autografts and allografts[7]
[8]
[9]
.
Calcium sulfate (CaSO4) has been used, researched, and reviewed
as
a
bone void filler for over 100 years, and has been
well-researched and thoroughly-reviewed by a many of
investigators [7,10,11,12,13]. In 1892, Dreesmann
reported on results from CaSO4 used to fill osseous
cavities in tuberculosis patients [14]. Since that
time, there have been numerous human clinical and animal
model-based reports on the effectiveness of CaSO4 as
a bone graft substitute in fields such as otolaryngology,
dentistry, and orthopedics,
animal models and clinical applications[15]. Although
the exact mechanism of action remains unknown, CaSO4
appears
to function as resorbable osteoconductive scaffold and space
filler, not only providing the structural framework necessary
for angiogenesis and osteogenesis but also preventing soft
tissue invasion
[16].
It is hypothesized that increased local acidity during CaSO4
resorption may result in demineralization of adjacent bone.
This demineralization would release matrix-bound morphogenetic
proteins that have a stimulatory effect on bone formation
[17].
Currently, surgical grade CaSO4 is available in
multiple forms such as Osteoset (Wright Medical Technology,
Inc., Arlington, TN), BonePlast (Interpore Cross, Irvine, CA),
Calceon 6 (Synthes, Paoli, PA), MIIG 115 (Wright Medical
Technology, Inc., Arlington, TN), MIIG X3 (Wright Medical
Technology, Inc., Arlington, TN), MIIG X3 HiVisc (Wright Medical
Technology, Inc., Arlington, TN). Among them, the recently
developed MIIG X3 (BCSC) series is designed to be injectable and
will harden in situ several minutes after injection with
high compressive strength. The authors report on the preliminary
result of this new bone graft substitute for the treatment of
periarticular fractures.
Materials
and Methods:
Sixty-one patients with periarticular fractures who were treated
with BCSC (Wright Medical Technology, Inc, Arlington, TN)
between October 2005 and
June 2008,
were included in this study. The patient population was
comprised of 41 males and 20 females whose mean age at surgery
was 52.3 years (range 26–77 years). The patients sustained
injuries in
36
road traffic accidents,
11
falls from height,
10
simple falls, and
4
sports injuries. According to fracture location, appropriate
preoperative radiographs were acquired in every patient (Fig.1A).
A computed tomography (CT) scan was acquired to determine the
displacement and extent of the depressed articular surface (Fig.1B).
The fracture location distribution is as follows:
4
proximal humeral fractures, 2 Neer type
Ⅲ,
2 Neer type
Ⅳ;
4
acetabular fractures, 2 posterior column and posterior wall
fractures, 2 posterior wall fractures ;
36
tibial plateau fractures, according to Schatzker classification,
24 Schatzker
Ⅱ,
7 Schatzker
Ⅲ,
1 Schatzker
Ⅳ,
2 Schatzker
Ⅴ,
2 Schatzker
Ⅵ;
7
distal tibial fractures, 4 Ruedi-Allgowe type
Ⅱ,
3 Ruedi-Allgowe type
Ⅲ;
10
calcaneal fractures, 4 Sanders type
Ⅱ,5
Sanders type
Ⅲ,1
Sanders type
Ⅳ.
Postoperative roentgenologic study was adapted to evaluate the
congruity of articular surface, bone replacement profile and
resorption process of bone substitute. The postoperative joint
function recovery was assessed with Neer’s score system
[18],
modified D’Aubigne and Postel hip scale
[19],
Rasmussen’s functional and anatomical grading score system
[20]
and Mazur ankle score
[21]
correspondingly to the shoulder, hip, knee and ankle joints .
The
Maryland Foot Score Profile
was
adapted for the postoperative evaluation of calcaneal fractures
[22].
Surgical
techniques
According to the fracture location, adequate anesthesia was
acquired. The fracture site was exposed and temporary reduction
was achieved with K-wires
and reduction clamps. The delivery needle was placed in the
deepest zone of the defect under fluoroscopic guidance. With the
use of the vacuum mixing machine, the dry CaSO4
powder and the solution were mixed. After mixing, the paste like
bone graft was placed in a syringe and injected into the
intraosseous void
with steady pressure. The injection of the BCSC was started from
the deepest recesses of the defect toward superficial areas by
withdrawing the canula in a retrograde fashion. With the
assistance of fluoroscopy, appropriate positioning of the graft
material was monitored in real time. Extra
caution
should be taken when treating severely comminuted fractures or
fractures with cartilage defects to avoid extravasation of the
graft material into the joint space. It’s not necessary to
remove the graft from the joint space or soft tissues since it
will be
resorbed
without affecting the local tissues.KELLY reference
The paste like graft materials (MIIG X3) must be injected within
3
minutes. The MIIG X3 HiVisc will be injectable up to
10 minutes. To prevent the contact between the blood and
the
graft material, which may interfere with the set time, after
injection the outer surface of the graft materials was covered
with dry gauze until it hardened completely. Complete set time
was about 9 -11 minutes after mixing began. For the tibial
plateau fractures, distal tibial fractures and acetabular
fractures, the injection of the graft was commonly performed
prior to definitive hardware placement and stabilization. Five
minutes after thorough hardening of the graft material, internal
fixation devices were applied with standard techniques. The
placement of screws across the hardened bone graft materials
provided increased screw purchase and intraoperative stability.
The thread of the screws should traverse the hardened graft
material to contralateral cancellous bone or penetrate the
contralateral cortex. Thus, the anti-pullout and support
strength of the screws was maintained throughout the process of
graft resorption and host bone replacement.
For proximal humeral fractures and calcaneal fractures, we
performed the internal fixation first.
Postoperative management
Proximal
humeral fractures.
The pendulum exercises are usually initiated on the second day
postoperative. Gently active, and assisted, range-of-motion
exercises are started approximately 2 weeks after the surgery
when the soft tissues healed. A sling is worn for 4 to 6 weeks.
Active motion is commenced after discontinuation of the sling.
Strengthening exercises are delayed until 12 weeks after
operation.
Tibial plateau
fractures.
Postoperatively the limb is placed
in a hinged brace. Continuous passive motion from full extension
to flexion of 30° is started on postoperative day 1. The rate
and the degree of flexion are increased as tolerated. Active
motion of the knee is initiated once the wound healed without
complications. Partial weight bearing of up to 50% of body
weight is begun at 8 to 10 weeks. Full weight bearing is not
allowed in 10-12 weeks until the follow-up x-rays show complete
union of fracture.
Distal tibial
fractures. The ankle is maintained in a
neutral position with a posterior plaster slab splint and the
lower extremity is elevated to reduce swelling. Active range of
motion of the ankle, subtalar joint, and foot/toes is initiated
on the second postoperative day. When the patient is not
exercising, the right-angled splint is applied again to prevent
equinus deformity. Partial weight bearing with the aid of
crutches is advanced at the 6 weeks. Usually the patients are
allowed to full weight bearing at 10-12 postoperative weeks with
the evidence of bone union.
Calcaneal
fractures.
The patient is placed in a well-padded soft bandage with a
posterior plaster slab at 90°. At the second postoperative week,
active range of motion of the ankle and subtalar joint is
instituted. No weight bearing is allowed for 8weeks. Minimal
toe-touch weight bearing with crutches is started at 8–10 weeks.
Full weight bearing is commenced at 10-12 weeks.
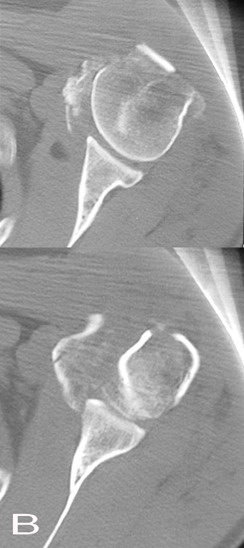
Fig. 1A and Fig. 1B: Preoperative anteroposterior
radiographs and axial CT scan revealing a Neer type
Ⅳ
fracture in left proximal hemurus
Results :
Fifty-seven of the 61 patients have sufficient follow up. One
proximal humeral fracture and 3 tibial plateau fractures were
lost postoperatively. The average length of follow-up was
20.2
months (range:
12-27months).
Postoperative radiographs were taken to assess the reduction of
the articular surface, bone healing
and the incorporation of bone graft
and new bone. Four week postoperative radiographs exhibited
resorption of the margin of the graft material compared with the
immediate
postoperative radiographs
(Fig.1C,
Fig.1D),
; however, no obvious radiolucent line was identifiable. The
graft material resorbed 67% (range:
50%-75%)
on the 8-week postoperative radiographs
with the formation of trabecula in
the
early absorption area (Fig.
1E).
Full bone graft incorporation was observed on the radiograph at
12-14 weeks postoperatively (Fig.1F).
In
young patients, the rate of bone-ingrowth
was
faster than that in elderly
patients. Six months
after operation, areas previously grafted demonstrated
equivalent bone density with the surrounding cancellous bone as
determined by radiographs. (Fig.1G).
There’s no significant difference of the
incorporation
rate between different sites
(Fig.1,Fig.2,
Fig.3).
Only two patients with tibial plateau fractures had
radiographically evident articular subsidence of 2mm at one year
after operation. Neither patient had joint dysfunction.
Fractures healed uneventfully in all the patients.
The average time for fracture healing is 8.2(range: 7-10) weeks
in proximal humeral fractures, 10.5(range: 9-12) weeks in
tibial plateau fractures,
12(range: 10-14) weeks in
distal tibial fractures and
11.3(range: 10-12) weeks in calcaneal
fractures. There were no infections. Wound exudations were
observed in two cases
of tibial plateau fractures. The cultures were taken and the
results
were negative. With empirical oral
antibiotics (Cephradine) and standard
dressing change,
the wounds
healed in 2-3 weeks.
According to Neer’s functional score system,
at
one year the postoperative score of three proximal humeral
fractures were
93, 93 and 91.
For the
4
acetabular fractures, the rating according to the Modified
D’Aubigne and Postal scale was excellent in 3 at one year and
good in 1
at
one year
follow-up.
For the thirty-three patients with tibial plateau fractures, the
postoperative knee function was good according to Rasmussen’s
functional and anatomical grading score system. Six months after
surgery,
the
mean function score was 25. 2 (range: 20-30) and the mean
fracture anatomic reduction score was 16.8 (range: 14-18)
in the 33 patients
available. One year after the operation, the thirty-three
patients’ mean function score reached 26.6(range: 24-30) and the
mean anatomic reduction score was 16.5(range: 14-18).
In the distal tibial fracture group, Mazur ankle scores were
excellent in 4, good in 2, and fair in 1 at six months
follow-up.
One year after surgery, ratings were excellent in excellent in
5
patients; good in
2
patients.
The Maryland Foot Score Profile was adapted to evaluate the
function recovery of the patients with calcaneal fractures. At
the 6 month follow-up timepoint, 7 patients had an excellent
rating with a mean score of 92.6 (range: 90-95), 2 patients had
a good rating with a mean score of 81.2 (range: 75-89), and one
patient had a fair rating with a score of 68. The one year
postoperative rating was excellent in all 8 patients with a mean
score of 93.4(range: 90-95), good rating was acquired in two
patients with a mean score of 84.5 (range: 75-89), fair rating
in one patients with a score of 71.
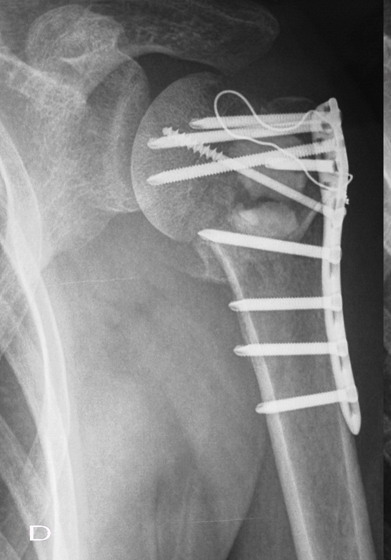
Fig. 1C : The radiopaque zone indicates the distribution
of graft material on the immediate postoperative anteroposterior
radiographs.
Fig. 1D : The 4-week postoperative radiograph exhibited
resorption of the margin of the graft material.
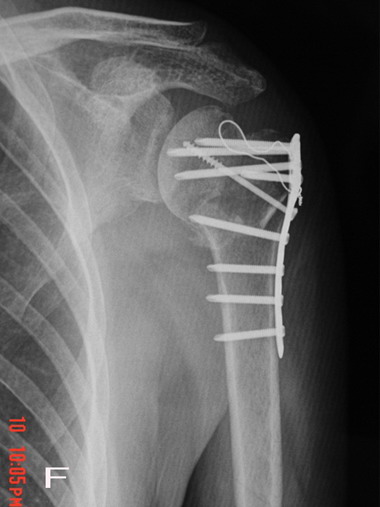
Fig. 1E : Nearly two third of the graft material was
absorbed at 8 weeks postoperatively on anteroposterior
radiograph.
Fig. 1F : Full bone graft incorporation was observed on
the radiograph at 12-14 weeks postoperatively.
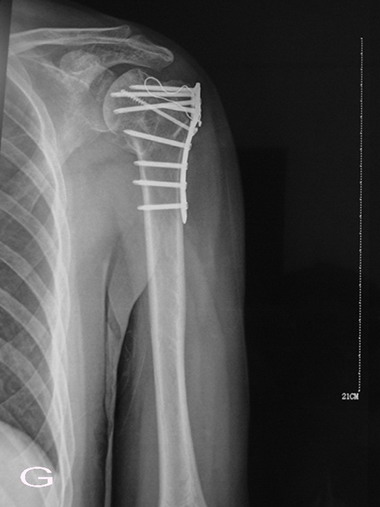
Fig. 1G: Six month after operation, radiograph
demonstrated equivalent bone density in the previous area of
graft material as surrounding cancellous bone.
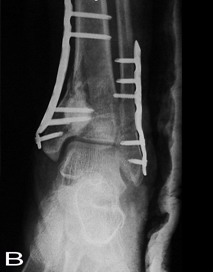
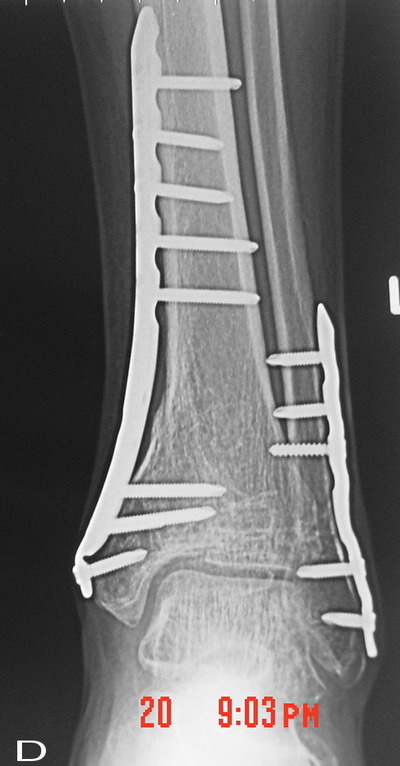
Fig. 2: The comparison of sequential radiographs at
follow-up the distal tibia showed no significant difference in
the incorporation rate of the graft material.
A: Preoperative, B: Postoperative, C:12 weeks after operation,
D: Six months after operation
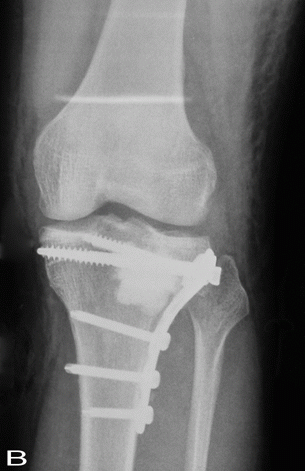
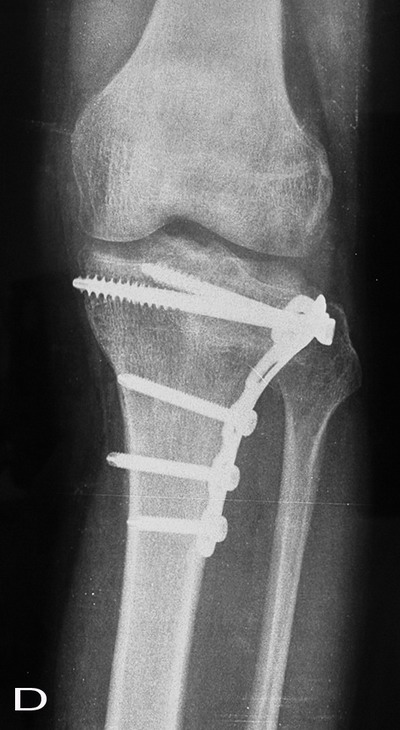
Fig. 3:
The comparison of sequential radiographs at follow-up tibial
plateau showed no significant difference in the incorporation
rate of the graft material.
A: Preoperative, B:
Postoperative, C:12 weeks after operation, D: Six months after
operation
Discussion :
For periarticular fractures, several issues need to be taken
into consideration. It is imperative that the articular surface
be anatomically reduced to decrease the chance of long term
complications such as pain or traumatic arthritis. In order to
avoid joint stiffness, adequate bone-implant construct strength
must be achieved to permit early rehabilitation. Periarticular
fracture internal fixation success, however, is always
challenged by two factors. The first factor is the subchondral
cancellous bone defect presented after elevation of depressed
articular fracture fragments. Filling of such defects
with a structural material is necessary to provide initial
mechanical support to the reduction and prevent subsidence of
the
articular surface during joint loading before bone healing. The
second factor is osteoporosis.
Insufficient screw purchase in weak osteoporotic bone increases
the risk of internal fixation failure and loss of reduction. In
osteoporotic patients, bone graft is especially important for
good fracture and hardware stabilization.
Bone grafts usually serve one or both of two main functions, as
a resource of osteogenetic cells and as a mechanical support.
[23] Autogenous bone graft with the ability of
osteogenesis and osteoconduction capabilities
is well known as the standard means of bone grafting despite the
limitations such as donor site morbidity and inadequate amount.
Autogeneous cancellous bone has greater potential for bone
formation and very limited structural strength as compared to
autogeneous cortical
bone. [24] Frozen cortical allograft bone is only
osteoconductive, and has the same structural support strength as
the autogenous cortical bone. [5] However, cortical
bone is very slow to incorporate.
[6,25,26]
Both
autograft and allograft share one drawback.
It is difficult to fill voids well in irregularly shaped defects
with both types of graft. Collectively, the drawbacks of
autograft and allograft materials impel scientists and medical
companies to develop additional bone graft substitute materials.
Most of the current available synthetic bone graft substitutes
can be grouped into hydroxyapatite products, soluble
calcium-based blocks and granules, or injectable cements.
[15] Among them, injectable cements with adequate
compressive strength that harden in situ have been used
to augment internal fixation during fracture surgery. The use of
Polymethylmethacrylate (PMMA) was recommended in several studies
to enhance fracture stability in the treatment of osteoporotic
fractures. [27] [28]. Because of its
exothermic curing,
and
inability to be absorbed and the risk of nonunion, PMMA is no
longer used in fracture treatment.
Injectable
calcium phosphate cements
have
been applied in the treatment of metaphyseal fractures for a
number of years. [8,29,30,31,32] [33] The
mechanical characteristics of calcium phosphate cements
vary in different forms. In general, cured calcium phosphate
cements have a compressive strength between that of cancellous
bone and cortical bone. Resorption of calcium phosphate cement
has been shown to occur very slowly. In a study by Kopylov et
al. calcium phosphate remained in the distal radius two
years after implantation. [30] Although Cassidy has
reported no adverse sequelae despite intra-articular extrusion
into the wrist joint, [34] the hardened calcium
phosphate cement may cause serious complications such as
traumatic arthritis in weight bearing joints. The calcium
phosphate cement presents some handling difficulties since the
internal fixation hardware must be placed before the injection
of graft materials, especially in severely comminuted fractures.[35]
An injectable biodegradable CaSO4 based bone graft
substitute, BCSC (Minimally Invasive Injectable Graft (MIIG)
Wright Medical Technology, Inc, Arlington, Tenn) was
manufactured from a surgical grade CaSO4 that is
uniform in shape and size, contrary to the plaster of Paris used
historically. The biodegradable cement was designed to harden
with the defect and provide intraoperative stabilization.
It
can be drilled through and receive self-tapping screws after
hardening without hindering material strength or integrity
properties. BCSC are recently developed products in
the
series. In vivo studies showed that the compression
strength of BCSC [11,36]may achieve 40MPa one hour
after preliminary set, and and reached levels approximately
equal to reported PMMA values after 24-48 hours.
[37] The adequate immediate stability and high
compressive strength is important to maintain the reduction and
prevent subsidence of the articular surface.
Watson reported
on
the use of
an
injectable CaSO4 in the treatment of five patients
with tibial plateau fracture and three patients with tibial
pilon fractures. All patients had excellent outcomes. In these
patients, 90%-100% absorption of the graft materials was
observed by 12 weeks postoperatively.
Three
patients sustained extravasation of graft material into the
joint space. These intra-articular materials were completely
absorbed
at
an average length of 15 days. They conclude the injectable CaSO4
was sufficient for intraoperative support and screw placement.
[13] In our study,
a
graft material with higher compressive strength BCSC (MIIG X3,
WMT, ArlintonTN) was used in the periarticular factures.
According to the Rasmussen’s knee functional score system and
the
Mazur ankle score system, the weight bearing joint function
in this series was good. Only two patients with tibial plateau
fractures had the articular subsidence of 2mm without joint
dysfunction,
indicating that the postoperative reduction was well maintained
from initial hardening to complete incorporation of the graft.
The incorperation rate of the graft material was similar between
the proximal tibia and distal tibia, which may imply that graft
remodeling is predictable and mainly determined by cement
microstructure rather than defect location. [13]
[10]
Moed et al reported on the use of CaSO4
pellets (Osteoset, WMT, Arlington, TN) in 32 patients with
acetabular fractures
who had intraarticular comminution or marginal impaction.
Follow-up CT scans showed good (>90%) bone ingrowth in 22 of the
31 patients studied. However, five patients had <50% bone
ingrowth, including one in whom there was no bone formation. The
authors theorized that synovial fluid contact was responsible
for pellet resorption without bone formation in these cases
since the pellets were in direct communication with the joint
space.
[11] In our study, good bone ingrowth was observed in
all four acetabular fracture cases. This may be attributed to
better void-filling characteristics of injectable CaSO4
cement over CaSO4 pellets in irregularly formed
defects. The small population of patients with acetabular
fractures in this series could be another factor.
In our opinion, this injectable calcium sulfate-based bone graft
substitute with high compressive strength is a promising
alternative for the treatment of periarticular fractures. The
utility of this material is of particular interest in
weight-bearing joints where reduction maintenance and an ability
to fill irregularly shaped defects are
important for great prognosis.
Acknowledgements:
I would like to recognize and thank Dr Ross M Wilkins who has
come through our paper over the past several months. His
revisions and suggestions are extremely valuable that make this
thesis could have reached its present form. Without his help and
support, this project would not have been possible.
Reference :
-
Arrington ED, Smith WJ, Chambers HG, et al. Complications of
iliac crest bone graft harvesting. Clin Orthop Relat Res,
1996(329): 300-309.
-
Goulet JA, Senunas LE, DeSilva GL, et al. Autogenous iliac
crest bone graft. Complications and functional assessment.
Clin Orthop Relat Res, 1997(339): 76-81.
-
Tomford WW. Transmission of disease through transplantation of
musculoskeletal allografts. J Bone Joint Surg Am, 1995,
77(11): 1742-1754.
-
Conrad EU, Gretch DR, Obermeyer KR, et al. Transmission of the
hepatitis-C virus by tissue transplantation. J Bone Joint Surg
Am, 1995, 77(2): 214-224.
-
Boyce T, Edwards J, Scarborough N. Allograft bone. The
influence of processing on safety and performance. Orthop Clin
North Am, 1999, 30(4): 571-581.
-
Wheeler DL, Enneking WF. Allograft bone decreases in strength
in vivo over time. Clin Orthop Relat Res, 2005(435): 36-42.
-
Kelly CM, Wilkins RM, Gitelis S, et al. The use of a surgical
grade calcium sulfate as a bone graft substitute: results of a
multicenter trial. Clin Orthop Relat Res, 2001(382): 42-50.
-
Goodman SB, Bauer TW, Carter D, et al. Norian SRS cement
augmentation in hip fracture treatment. Laboratory and initial
clinical results. Clin Orthop Relat Res, 1998(348): 42-50.
-
Shors EC. Coralline bone graft substitutes. Orthop Clin North
Am, 1999, 30(4): 599-613.
-
Kelly CM, Wilkins RM. Treatment of benign bone lesions with an
injectable calcium sulfate-based bone graft substitute.
Orthopedics, 2004, 27(1 Suppl): s131-135.
-
Moed BR, Willson Carr SE, Craig JG, et al. Calcium sulfate
used as bone graft substitute in acetabular fracture fixation.
Clin Orthop Relat Res, 2003(410): 303-309.
-
Turner TM, Urban RM, Gitelis S, et al. Radiographic and
histologic assessment of calcium sulfate in experimental
animal models and clinical use as a resorbable bone-graft
substitute, a bone-graft expander, and a method for local
antibiotic delivery. One institution's experience. J Bone
Joint Surg Am, 2001, 83-A Suppl 2(Pt 1): 8-18.
-
Watson JT. The use of an injectable bone graft substitute in
tibial metaphyseal fractures. Orthopedics, 2004, 27(1 Suppl):
s103-107.
-
Dressmann H. Ueber Knochenplombierung bei Hohlenformigen
Defekten des Knochens. Beitr Klin Chir, 1892, 9804+810.
-
Bauer TW, Togawa D. Bone graft substitutes: towards a more
perfect union. Orthopedics, 2003, 26(9): 925-926.
-
Peters CL, Hines JL, Bachus KN, et al. Biological effects of
calcium sulfate as a bone graft substitute in ovine
metaphyseal defects. J Biomed Mater Res A, 2006, 76(3):
456-462.
-
Sidqui M, Collin P, Vitte C, et al. Osteoblast adherence and
resorption activity of isolated osteoclasts on calcium
sulphate hemihydrate. Biomaterials, 1995, 16(17): 1327-1332.
-
Neer CS, 2nd. Displaced proximal humeral fractures. I.
Classification and evaluation. J Bone Joint Surg Am, 1970,
52(6): 1077-1089.
-
Charnley J. The long-term results of low-friction arthroplasty
of the hip performed as a primary intervention. J Bone Joint
Surg Br, 1972, 54(1): 61-76.
-
Rasmussen PS. Tibial condylar fractures. Impairment of knee
joint stability as an indication for surgical treatment. J
Bone Joint Surg Am, 1973, 55(7): 1331-1350.
-
Mazur JM, Schwartz E, Simon SR. Ankle arthrodesis. Long-term
follow-up with gait analysis. J Bone Joint Surg Am, 1979,
61(7): 964-975.
-
Sanders R, Fortin P, DiPasquale T, et al. Operative treatment
in 120 displaced intraarticular calcaneal fractures. Results
using a prognostic computed tomography scan classification.
Clin Orthop Relat Res, 1993(290): 87-95.
-
Goldberg VM, Stevenson S. Natural history of autografts and
allografts. Clin Orthop Relat Res, 1987(225): 7-16.
-
Parikh SN. Bone graft substitutes in modern orthopedics.
Orthopedics, 2002, 25(11): 1301-1309; quiz 1310-1301.
-
Enneking WF, Eady JL, Burchardt H. Autogenous cortical bone
grafts in the reconstruction of segmental skeletal defects. J
Bone Joint Surg Am, 1980, 62(7): 1039-1058.
-
Burchardt H, Enneking WF. Transplantation of bone. Surg Clin
North Am, 1978, 58(2): 403-427.
-
Bartucci EJ, Gonzalez MH, Cooperman DR, et al. The effect of
adjunctive methylmethacrylate on failures of fixation and
function in patients with intertrochanteric fractures and
osteoporosis. J Bone Joint Surg Am, 1985, 67(7): 1094-1107.
-
Schatzker J, Ha'eri GB, Chapman M. Methylmethacrylate as an
adjunct in the internal fixation of intertrochanteric
fractures of the femur. J Trauma, 1978, 18(10): 732-735.
-
Sanchez-Sotelo J, Munuera L, Madero R. Treatment of fractures
of the distal radius with a remodellable bone cement: a
prospective, randomised study using Norian SRS. J Bone Joint
Surg Br, 2000, 82(6): 856-863.
-
Kopylov P, Runnqvist K, Jonsson K, et al. Norian SRS versus
external fixation in redisplaced distal radial fractures. A
randomized study in 40 patients. Acta Orthop Scand, 1999,
70(1): 1-5.
-
Schildhauer TA, Bauer TW, Josten C, et al. Open reduction and
augmentation of internal fixation with an injectable skeletal
cement for the treatment of complex calcaneal fractures. J
Orthop Trauma, 2000, 14(5): 309-317.
-
Horstmann WG, Verheyen CC, Leemans R. An injectable calcium
phosphate cement as a bone-graft substitute in the treatment
of displaced lateral tibial plateau fractures. Injury, 2003,
34(2): 141-144.
-
Simpson D, Keating JF. Outcome of tibial plateau fractures
managed with calcium phosphate cement. Injury, 2004, 35(9):
913-918.
-
Cassidy C, Jupiter JB, Cohen M, et al. Norian SRS cement
compared with conventional fixation in distal radial
fractures. A randomized study. J Bone Joint Surg Am, 2003,
85-A(11): 2127-2137.
-
Lobenhoffer P, Gerich T, Witte F, et al. Use of an injectable
calcium phosphate bone cement in the treatment of tibial
plateau fractures: a prospective study of twenty-six cases
with twenty-month mean follow-up. J Orthop Trauma, 2002,
16(3): 143-149.
-
Beardmore AA, Brooks DE, Wenke JC, et al. Effectiveness of
local antibiotic delivery with an osteoinductive and
osteoconductive bone-graft substitute. J Bone Joint Surg Am,
2005, 87(1): 107-112.
-
Belkoff SM, Sanders JC, Jasper LE. The effect of the
monomer-to-powder ratio on the material properties of acrylic
bone cement. J Biomed Mater Res, 2002, 63(4): 396-399.
|